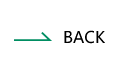
Charge-Transferonics
source:admin update time:2025-03-19 13:03:59


Researchers have long been seeking artificial and controllable methods for fabricating superlattices to enable band engineering of the composite 2D electron gas above them. Examples include self-assembled nanospheres, micro/nanofabricated nanoscale channels, or hole arrays. However, to date, the band modulation effects achievable with these artificial superlattices remain limited due to constraints such as microfabrication precision.
One may thus pose a fundamental question: Is there a method that can bypass the limitations of moiré patterns in solid-state materials and, from the perspective of Coulomb interactions, construct a "quantum superlattice" with tunable correlation strength in situ, thereby enabling a richer and more diverse range of physical phenomena?
Notably, charge density waves (CDWs) or Wigner crystals, which form periodic spatial lattices with wavelengths ranging from nanometers to tens of nanometers, seem like intuitive candidates for such explorations.
Recent research has revealed that in a vertical heterostructure composed of monolayer graphene and chromium oxychloride (CrOCl) (Figure 1), a quasi-2D interfacial electronic state may exist—potentially forming what can be considered a quantum superlattice. This interfacial state is primarily localized within the Cr atomic layer, approximately 0.7 nm beneath the graphene layer (Figure 1c).
Theoretically, these interfacial electrons can undergo spontaneous lattice translational symmetry breaking due to their own correlation effects, leading to the formation of long-range charge order with wavelengths ranging from a few nanometers to tens of nanometers. This long-range superperiodicity further enhances the electron-electron interactions within graphene, significantly increasing the Fermi velocity of Dirac electrons near the charge neutrality point and inducing a bandgap opening (Figure 1b).
When subjected to an external vertical electric field, once the Fermi level of the interfacial state aligns with that of graphene, a portion of the charge carriers that would otherwise populate graphene can be transferred to the interfacial state via tunneling. Once these carriers enter the CrOCl interfacial state, they undergo Wigner crystallization, forming an insulating superlattice composed of long-range ordered electrons.
Under this mechanism, the dual-gate control Landau fan diagram of the sample in a magnetic field exhibits significant distortions in certain parameter regions, as shown in Figure 1d.
Fig. 1. Band structure reconstruction induced by interfacial charge orderings.
Notably, in this interfacially coupled quantum Hall state, the quantization of transverse conductance can occur at exceptionally low magnetic fields and remains robust even above liquid nitrogen temperature. For example, at 77 K, the system achieves a well-defined quantum Hall plateau at a filling factor of ±2 with a magnetic field as low as 0.35 T— a record-low value that can be easily provided by a conventional permanent magnet. In contrast, conventional graphene requires a magnetic field exceeding 10 T to achieve quantized conductance at 77 K.
This significant reduction in the required magnetic field marks a crucial step in extending the applicability of quantum Hall edge states from the liquid helium temperature regime to the liquid nitrogen regime, paving the way for future applications in topological superconductivity, quantum Hall Fabry-Pérot interferometry, and other advanced quantum electronic devices.
